the Creative Commons Attribution 4.0 License.
the Creative Commons Attribution 4.0 License.
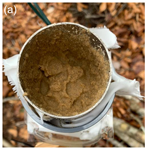
Short communication: Concentrated impacts by tree canopy drips – hotspots of soil erosion in forests
Ayumi Katayama
Kazuki Nanko
Seonghun Jeong
Tomonori Kume
Yoshinori Shinohara
Steffen Seitz
The degradation of ground vegetation cover caused by large grazing herbivores frequently results in enhanced erosion rates in forest ecosystems. Splash erosion can be caused by drop impacts with a high throughfall kinetic energy (TKE) from the tree canopy. Notably larger canopy drips from structurally mediated woody surface points appear to induce even higher TKE and generate concentrated impact locations causing severe focus points of soil erosion. However, TKE at these locations has rarely been reported. This pilot study investigated the intensity of TKE at a concentrated impact location and compared it with general TKE locations under the canopy and freefall kinetic energy (FKE) outside the forest. We measured precipitation, TKE and FKE using splash cups at seven locations under Japanese beech trees and five locations outside the forest during the leafless and leafed seasons in 2021 in a mixed forest with evergreen coniferous trees and deciduous broadleaved trees in Japan. The TKE at the concentrated impact location was 15.2 and 49.7 times higher than that at the general locations under the beech and FKE, respectively. This study confirmed that canopy drip from woody surfaces could be a hotspot of soil erosion in temperate forest ecosystems. Throughfall precipitation at the concentrated impact location was 11.4 and 8.1 times higher than that at general locations and freefall, respectively. TKE per 1 mm precipitation (here, “unit TKE”) at the concentrated impact location (39.2 ± 23.7 J m−2 mm−1) was much higher than that at general locations (22.0 ± 12.7 J m−2 mm−1) and unit FKE (4.5 ± 3.5 J m−2 mm−1). Unit TKE in the leafless season was significantly lower than in the leafed season because of fewer redistribution of canopy drips induced only by woody tissue. Nevertheless, unit TKE at the concentrated impact location in the leafless season (36.4 J m−2 mm−1) was still higher than at general locations in the leafed season. These results show that potentially high rates of sediment detachment can be induced not only by throughfall precipitation but also by larger throughfall drop size distributions at the concentrated impact location, even in the leafless season. Further studies with more replication building on this first report are necessary to investigate how many of these concentrated impact locations may occur on average with different tree species to better assess the extent of the erosion risk under forests.
- Article
(2952 KB) - Full-text XML
-
Supplement
(402 KB) - BibTeX
- EndNote
Soil conservation is an important environmental challenge of the 21st century as soils are the foundation of life and a reservoir for water, carbon and nutrients (Lal, 2014). The threat to soil composition is evident worldwide, especially in areas with regularly recurring extreme climatic events such as heavy rainfall (Borrelli et al., 2020). Soil erosion rates induced by water are mainly determined by rainfall patterns such as raindrop kinetic energy and ground cover by vegetation (Seitz et al., 2017). Severe soil erosion events are rare in forest ecosystems because the general abundant ground cover owing to understory vegetation or plant litter (Miura et al., 2003; Holz et al., 2015). Therefore, forests can be seen as one of the most effective land use types to mitigate soil losses (Pimentel and Burgess, 2013). However, disturbance of forest vegetation may lead to significant small-scale (Gall et al., 2022; Geißler et al., 2010) and areal (Safari et al., 2016; Seitz et al., 2016; Zemke et al., 2016) erosion events that can by far exceed sustainable erosion rates (Deng et al., 2023). Important examples have been described globally such as in Hungary (Misik and Kárász, 2022) and China (Yao et al., 2019). In Japan in particular, understory vegetation in forests is regularly damaged by sika deer (Cervus nippon) (Murata et al., 2009; Takatsuki, 2009). The degradation of protective vegetation layers frequently results in enhanced splash erosion through direct raindrop impacts and increased surface runoff with significant erosion potential (Shinohara et al., 2018; Song et al., 2019).
Throughfall kinetic energy (TKE, in J m−2) can be determined from drop size and velocity in addition to the precipitation amount. TKE has partly been shown to be higher than freefall kinetic energy (FKE) outside vegetation layers. This phenomenon is attributed to the capacity of the forest canopy to generate large new canopy drips after the first interception, which depends on the species (Chapman, 1948; Nanko et al., 2015). Canopy drip can contribute to more than half of the total throughfall volume from leafed canopies (Levia et al., 2019). In canopy water flow, the lateral redistribution plays an important role in creating local concentrations of throughfall (Keim and Link, 2018). Subsequently, lateral canopy water flow paths ending at structurally mediated woody surface drip points, such as irregular rough points and branch concavities, accumulate more water volume transported down the branch with a longer residence time and then generate larger diameter drops in greater volumes (Nanko et al., 2022) than foliar surfaces (Levia et al., 2019; Nanko et al., 2016, 2022). Notably larger canopy drips can have higher TKE and therefore generate concentrated impact locations, potentially causing severe soil erosion. However, the TKE at these concentrated impact locations and the subsequent splash erosion potential have rarely been described in the literature and have not yet been quantified.
TKE is linearly correlated with throughfall precipitation in monolayer coniferous forests (Shinohara et al., 2018). The slope of the relationship between throughfall precipitation and TKE is known as unit TKE, that is, TKE per 1 mm precipitation. The unit TKE differs with canopy species and architecture as well as rainfall intensity (Nanko et al., 2013, 2015; Liu et al., 2022). Throughfall from woody surface drip points consist of larger canopy drips, suggesting that the unit TKE at such concentrated impact locations is different from that at other general locations. Furthermore, this relationship might also differ between the leafed and leafless seasons, owing to the difference in the distribution of drops of different sizes (Levia et al., 2017). Thus, TKE can considerably affect soil erosion rates also in the leafless season when the contribution of drip points to the total throughfall precipitation becomes dominant (Levia et al., 2019). Therefore, knowledge of the significance of TKE at concentrated impact locations and seasonal changes in TKE in response to leaf status is vital for understanding soil erosion risk in forests with degraded ground cover.
This pilot study investigated TKE under broadleaved trees in Shiiba Research Forest, Kyushu, Japan, which is a substantially disturbed and eroded forest ecosystem caused by deer grazing. A special focus of this work is given to unusual high energy levels induced by structurally mediated woody surface drip points which partly occurred during the measurement campaign to estimate throughfall erosivity. We quantified the TKE intensity at the concentrated impact location. We hypothesized that (1) unit TKE at the concentrated impact location is higher than that at general locations inducing elevated splash erosion and (2) the relationship between throughfall precipitation and TKE differs with the leaf status of trees.
2.1 Study site
This study was conducted in Shiiba Research Forest, Kyushu, southern Japan (32∘40′ N, 131∘17′ E, 1030 m a.s.l.). The study site includes a mixed forest with evergreen coniferous trees and deciduous broadleaved trees. The mean annual temperature and precipitation were 10.8 ∘C and 3278 mm, respectively, as measured at a meteorological station located 3 km from the study site at 1180 m a.s.l. Monthly precipitation in March, April, August, September and August of 2021 was 162, 133.5, 958.5, 170 and 41.5 mm at the University Forest office, situated 4 km away from the study site (600 m a.s.l.). Formerly, this area was characterized by a dense understory vegetation comprised primarily of bamboo (Sasa borealis [Hack.] Makino & Shibata). However, this understory vegetation has mostly disappeared since around the year 2000, coinciding with a documented rise in the Sika deer population. Currently, there is no intact understory vegetation in most areas of the research forest (Kawakami et al., 2020). Therefore, distinct erosion forms and root exposure can be widely observed (Katayama et al., 2023) and soil degradation has been identified a major challenge for forest services (Abe et al., 2022).
2.2 Throughfall kinetic energy
TKE and FKE were determined using splash cups (Shinohara et al., 2018; Scholten et al., 2011). The splash cups are filled with a standardized sand and weighed dry before deployment in the field. Subsequently, raindrops hit the sand surface and detached sand is partly splashed away from the cup. The loss of sand (LoS, g m−2) was measured by back weighing remaining dried sand volumes and subtracting the amount from the initial amount. Kinetic energy (KE, in J m−2) can be estimated from its relationship with LoS using a linear function (KE = 14.55 × LoS, Scholten et al., 2011). This method has proven to be reliable and cost efficient with a high number of replications (Geißler et al., 2012) and is suitable to evaluate spatial variation in TKE (Shinohara et al., 2018). We used the splash cups with the diameter of 5.0 cm, height of 5.1 cm and volume of 100 cm3. These are slightly larger than those reported by Scholten et al. (2011) (4.6 cm in diameter and 3.6 cm in height, respectively) but accurately estimated TKE by using a linear equation (Shinohara et al., 2018).
The LoS was measured during each of the five rainfall events in the leafless (March to April) and leafed (August to September) seasons of 2021. Seven splash cups were installed under the canopy of two Fagus crenata trees for TKE (Fig. 2). One position was chosen at a possible concentrated drip location formed by a structurally mediated wood surface and where more throughfall precipitation was visually observed during rainfall events. Six more splash cups at different positions under the canopy were installed to measure TKE at general locations. Five splash cups were further installed outside the forest to measure FKE, which were 40 m apart from the locations under the canopy. A storage-type bottle with a funnel (diameter: 9.0 cm) was installed next to each splash cups to measure precipitation. Precipitation was measured at the same time with TKE measurement. The distance between the splash cup and precipitation collector was about 5 cm.
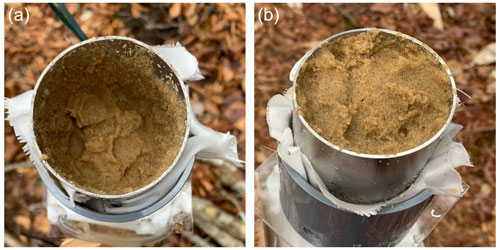
Figure 1Splash cups at the concentrated impact location (a) and at an exemplary general location (b) after the first rainfall event in the leafless season. Freefall precipitation of this event was 35.4 mm.
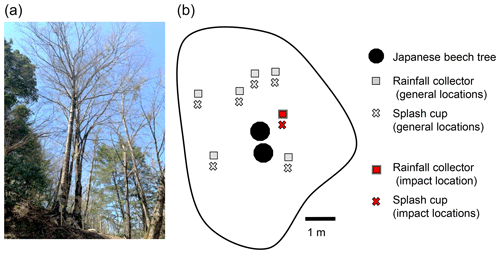
Figure 2Japanese beech trees studied in this study (a). The splash cups and rainfall collectors were installed under the beech trees (b). The black line shows canopy projected area. The splash cups and rainfall collectors outside the forest were installed 40 m apart from the trees.
At the concentrated impact location, the collection of LoS and throughfall precipitation failed during some very strong rainfall events during the leafed period. We obtained data of 10 events at the general locations (Table 1), but TKE and throughfall precipitation at the impact location were obtained only for 7 and 6 events. Thus, the relationship between TKE and freefall precipitation (TKE = 237.1 × freefall precipitation, R2=0.92) was established using the data obtained over seven events, whereas the relationship between throughfall precipitation and freefall precipitation (throughfall precipitation = 8.23 × freefall precipitation, R2=0.97) was established using the data obtained over six events.
Table 1Event-scale precipitation, kinetic energy and unit kinetic energy at the impact location and general locations under Japanese beech trees and outside the forest in the leafless and leafed seasons in 2021, respectively.
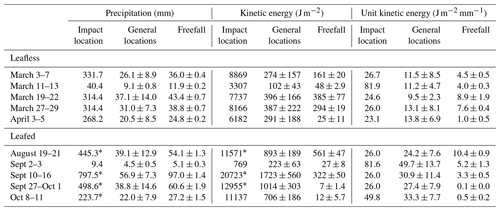
Data are given as mean ± standard deviation. * The data were estimated from freefall precipitation.
2.3 Tree traits
Diameter at breast height of the two selected beech trees was 46.0 and 46.1 cm, and tree height was 21.1 and 18.0 m, respectively. Leaf area index (LAI) was determined using a single reflex camera system with a fish eye lens (THETA SC; Ricoh Co. Ltd., Tokyo, Japan) and software (Gap Light Analyzer version 2.0, Frazer et al., 1999) to be 4.5 and 0.9 at the concentrated impact location in the leafed and leafless season, respectively. LAI at general locations ranged from 1.7 to 4.9 with a mean of 3.3 in the leafed season and from 0.1 to 0.6 with a mean of 0.3 in the leafless season. Branch height at the concentrated impact location was 9.1 m and ranged from 6.5 to 13.5 m at the six splash cup positions with an average of 9.1 m. Average leaf area and leaf mass per area obtained from beech leaves in our study forest were 10.5 cm2 and 84.7 g m−2, respectively. The bark of the beech was smooth; however there was moss cover in some places along the stem and epiphytic moss at the base of the branch, from which considerable amounts of water dropped on the ground.
2.4 Statistical analysis
The significant difference in the slopes of the relationships of throughfall precipitation with TKE between concentrated impact location and general locations was examined using analysis of covariance (ANCOVA, P<0.05). The significant difference in slopes in the relationships between the leafed and leafless seasons was examined separately for impact and general locations (ANCOVA, P<0.05). In these analyses, TKE data that were not measured in the three rainfall events were excluded. Intercepts were set to zero for the models. All statistical analyses were performed using R software version 3.6.2 (R Core Team, 2019).
3.1 Effect of structurally designed high energy points on TKE
Considerably high TKE was observed at the concentrated impact location under the beech (Fig. 1). This location received a focused number of canopy drips from an overlying structurally mediated woody surface drop point (see “Video supplement”). The average ± SD of TKE at the concentrated impact location (9142 ± 5522 J m−2) for all seasons was 15.2 times higher than at general locations under the beech (601 ± 495 J m−2) and 49.7 times higher than FKE (184 ± 195 J m−2, Table 1). The average of throughfall precipitation at the concentrated impact location (324 ± 227 mm) was 11.4 times higher than that at general locations under beech (29 ± 16 mm) and 8.1 times higher than that from freefall precipitation (40 ± 26 mm).
Across all rainfall events, TKE significantly increased with throughfall precipitation at both the concentrated impact location and general locations regardless of canopy leaf conditions (Fig. 3). It could be shown that TKE at the concentrated impact location was higher than at general locations, with a significant difference in the relationships between TKE and throughfall precipitation (Fig. 3). Thus, the first hypothesis can be confirmed. Furthermore, the branch height at the concentrated impact location was comparable to average of branch height at other general drip points, indicating that higher unit TKE was mostly induced by larger drop sizes. Note that the unit TKE is determined from raindrop size distributions and canopy height when the canopy height is less than the height for the rain-drop terminal velocity (Shinohara et al., 2018). Previous study showed that most canopy drips did not reach terminal velocity where the mean first living branch height was 7.9 m (Nanko et al., 2008). Raindrops with diameters >3 mm need at least a 12 m fall distance to gain terminal velocity (Wang and Pruppacher,1977). Although the branch height could be one factor determining TKE in the present study, considerably higher TKE at the impact location was not caused by the height because of the comparable branch height. Thus, the TKE at the concentrated impact locations originating from woody surfaces was induced by both high throughfall precipitation and large drop size, which is an important cause of splash erosion and might be considered an underestimated hotspot of sediment translocation.
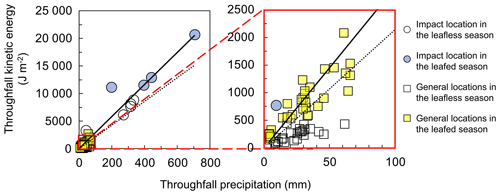
Figure 3Relationship between event-based throughfall precipitation and event-based throughfall kinetic energy (TKE). Circles and squares show TKE measured at each concentrated impact location and each TKE at general locations, respectively. Closed and open symbols show leafless and leafed seasons. Solid and dotted lines show the regression lines at the concentrated impact location and general locations, respectively. The relationships were significantly different between the locations (ANCOVA, P<0.01).
3.2 Effects of leaf status
In the leafed season, the event-scale average TKE at the concentrated impact location was 12.5 times higher than that at general locations under the beech tree and 61.5 times higher than FKE (Table 1). The event-scale mean throughfall precipitation at the concentrated impact location was 12.2 times higher than at general locations and 8.1 times higher than freefall precipitation. In the leafless season, the average TKE at the concentrated impact location was 23.6 times higher than that at general locations and 37.6 times higher than FKE, whereas mean throughfall was 10.3 times higher at general locations and 8.2 times higher than freefall precipitation. These results suggest that the splash erosion risk at the impact location remained high in the leafless season although the risk was lower than that in the leafed season. The ratios of throughfall precipitation at the concentrated impact location and general locations compared to freefall precipitation were 8.1 and 0.71, respectively, suggesting that throughfall precipitation decreased with canopy interception, whereas the identified hotspot of throughfall selectively increased it. The slopes of the relationships between TKE and throughfall precipitation at the concentrated impact location and general locations were higher in the leafed season than in the leafless season (ANCOVA, P<0.01). Therefore, we can conclude that unit TKE strongly increases with the presence of leaves and potential splash erosion is higher during the leafed period. However, unit TKE at the concentrated impact location in the leafless season (36.4 J m−2 mm−1) was still higher than at general locations in the leafed season (32.1 ± 10.3 J m−2 mm−1). This suggests high splash erosion risk at the concentrated impact location even in the leafless season. In summary, leaf status has been shown to generate a distinct impact and differential effects; therefore, the second hypothesis can be accepted.
Additionally, the differences between TKE and FKE, as well as throughfall and freefall precipitation, appeared to be less pronounced in the leafless season. Levia et al. (2019) showed that canopy drips under broadleaved trees accounted for 69 % of the total throughfall precipitation in the leafed phenophase, compared to 8 % in the leafless phenophase. Most of the throughfall at general locations under leafless trees were freefall. The soil erosion risk is lower during the leafless season than during the leafed season except for the concentrated drop impact locations.
3.3 Implication and uncertainty
This study remarked notably high TKE under investigated beech trees. Mean unit FKE was reported by van Dijk et al. (2002) as 14.2, 18.6, 26.5 and 28.1 J m−2 mm−1 with rainfall rates of 1, 10, 50 and 100 mm h−1, respectively. The maximum measured unit FKE was 28.3 J m−2 mm−1. As for throughfall, unit TKE reported in previous studies ranged from 16.4 to 28.1 J m−2 mm−1 in Japan (Nanko, 2013), Hawaii (Nanko et al., 2015) and Thailand (Nanko et al., 2020). The unit TKE at the concentrated impact location in the present study was much higher than these previously reported values, suggesting that higher throughfall precipitation and larger throughfall drop size distributions can result in an increased risk of soil erosion. Furthermore, unit TKE for general locations in the present study was also higher than in previously measured Japanese cypress plantations where it was 16.4–21.0 J m−2 mm−1 (Nanko, 2013). The median volume drop size of canopy drip from leaves was 4.7 mm in Japanese cypress but 5.2 mm in beech (Nanko et al., 2013). This difference was caused by various leaf traits such as leaf area, leaf shape and leaf surface water repellency (Levia et al., 2017). Thus, TKE generation is strongly species specific and TKE under beech trees may be higher than that under other tree species.
Finally, although considerably higher TKE at the concentrated impact location was measured using splash cups, it should be noted that TKE at the concentrated impact location in the present study may have been underestimated due to the rim effect related to the splash cup measuring system. There is some uncertainty in the estimated TKE if sand particles are starting to hit the cup wall instead of flying out. This phenomenon occurred particularly at the concentrated impact location. Thus, TKE at the concentrated impact location may be even higher than that reported in the present study.
This pilot study showed a possible increase in soil erosion risk under the forest canopy using a limited dataset obtained at one impact location. TKE and throughfall precipitation can considerably vary with tree traits; thus, it is necessary to clarify spatial variations in TKE and throughfall and factors determining variations such as tree traits and leaf status based on random sampling with more replication in future studies. Regarding the erosion potential, it is furthermore of high importance to investigate how many of these concentrated impact locations may occur on average with different tree species to better assess the general extent of the erosion risk.
In this paper, we report the results of a pilot splash cup experiment conducted to investigate potential erosion from one high energy water release point under the canopy in a disturbed Japanese forest environment. Strongly enhanced TKE was observed from structurally mediated woody surface points under beech (Fagus crenata), which was approximately 15 times higher than that at general locations and approximately 50 times higher than FKE. The higher kinetic energy was caused by both higher throughfall precipitation and greater unit kinetic energy. These results underline the evidence of high soil erosion risk in forested areas owing to particular tree traits and show that this risk can significantly exceed the previously known dimensions at specific points under the tree canopy. Moreover, unit TKE at high-energy and general locations was reduced in the leafless season, but in the leafless season it was still higher at the concentrated impact location than at general locations in the leafed season. This result points to a potentially enhanced soil erosion risk even outside the growing season if concentrated impact locations with high kinetic energies occur in larger numbers on trees. Precipitation amount is the most important factor determining soil erosion risk; more precipitation also results in severe erosion risk in the leafed season. Further research is necessary to verify the results, expanding them to include other tree species and forest ecosystems, and to shed light on the mechanistic effects of distinct plant characteristics, building upon this pilot study. In this context, it should also be investigated how many of these concentrated impact locations may occur on average with different tree species to better assess the extent of soil erosion. This becomes particularly important when the protective soil cover layer of understory or leaf litter is disturbed or removed. Therefore, future studies examining soil erosion rates under forests need to considerate both changes in TKE through plant traits and variations in ground cover. Finally, as this pilot study showed considerable high soil erosion risk using a dataset obtained at one single impact location, more measurements under different trees with more replication are needed.
All raw data are provided in the Supplement.
The video supplement is available at the website https://doi.org/10.5446/61199 (Katayama, 2023).
The supplement related to this article is available online at: https://doi.org/10.5194/esurf-11-1275-2023-supplement.
AK, KN and StS designed the experiment, AK, YS, TK and SJ carried it out and AK, KN and StS prepared the article with contributions from all co-authors.
The contact author has declared that none of the authors has any competing interests.
Publisher’s note: Copernicus Publications remains neutral with regard to jurisdictional claims made in the text, published maps, institutional affiliations, or any other geographical representation in this paper. While Copernicus Publications makes every effort to include appropriate place names, the final responsibility lies with the authors.
We thank the technical staff of Shiiba Research Forest, who helped with the preparation and establishment of measurements.
This study was financially supported by JSPS KAKENHI (grant no. 22H03793) and JSPS Postdoctoral Fellowship for Research in Japan (Short-term) (grant no. PE21018). We also acknowledge the Kyushu University Fund which allowed us to meet in Shiiba.
This paper was edited by Sagy Cohen and reviewed by two anonymous referees.
Abe, H., Fu, D., Kume, T., and Katayama, A.: Exposure of tree roots and its control factors in a mixed temperate forest with no understory vegetation, Bull. Kyushu Univ For., 103, 13–20, https://doi.org/10.15017/4776829, 2022 (Japanese with English summary).
Borrelli, P., Robinson, D. A., Panagos, P., Lugato, E., Yang, J. E., Alewell, C., Wuepper, D., Montanarella, L., and Ballabio, C.: Land use and climate change impacts on global soil erosion by water (2015–2070), P. Natl. Acad. Sci. USA, 117, 21994–22001, https://doi.org/10.1073/pnas.2001403117, 2020.
Chapman, G.: Size of raindrops and their striking force at the soil surface in a red pine plantation, Eos, Transactions American Geophysical Union, 29, 664–670, 1948.
Frazer, G. W., Canham, C. D., and Lertzman, K. P.: Gap Light Analyzer (GLA), Version 2.0: Imaging software to extract canopy structure and gap light transmission indices from true colour fisheye photographs, users manual and program documentation, Simon Fraser University, Burnaby, British Columbia, and the Institute of Ecosystem Studies, Millbrook, New York, 36, 1999.
Deng, J., Fang, S., Fang, X., Jin, Y., Kuang, Y., Lin, F., Liu, J., Ma, J., Nie, Y., Ouyang, S., Ren, J., Tie, L., Tang, S., Tan, X., Wang, X., Fan, Z., Wang, Q.-W., Wang, H., and Liu, C.: Forest understory vegetation study: current status and future trends, Forestry Research, 3, 6, https://doi.org/10.48130/FR-2023-0006, 2023.
Gall, C., Nebel, M., Quandt, D., Scholten, T., and Seitz, S.: Pioneer biocrust communities prevent soil erosion in temperate forests after disturbances, Biogeosciences, 19, 3225–3245, https://doi.org/10.5194/bg-19-3225-2022, 2022.
Geißler, C., Kühn, P., Shi, X., and Scholten, T.: Estimation of throughfall erosivity in a highly diverse forest ecosystem using sand-filled splash cups, J. Earth Sci., 21, 897–900, 2010.
Geißler, C., Kühn, P., Böhnke, M., Bruelheide, H., Shi, X., and Scholten, T.: Splash erosion potential under tree canopies in subtropical SE China, Catena, 91, 85–93, https://doi.org/10.1016/j.catena.2010.10.009, 2012.
Holz, D. J., Williard, K. W., Edwards, P. J., and Schoonover, J. E.: Soil erosion in humid regions: A review, Journal of Contemporary Water Research & Education, 154, 48–59, 2015.
Katayama, A.: Structurally-mediated woody surface drip points for beech tree, TIB [video], https://doi.org/10.5446/61199, 2023.
Katayama, A., Oyamada, M., Abe, H., Uemori, K., and Hishi, T.: Soil erosion decreases soil microbial respiration in Japanese beech forests with understory vegetation lost by deer, J. For. Res., 28, 428–435, https://doi.org/10.1080/13416979.2023.2235499, 2023.
Kawakami, E., Katayama, A., and Hishi, T.: Effects of declining understory vegetation on leaf litter decomposition in a Japanese cool-temperate forest, J. For. Res., 25, 260–268, 2020.
Keim, R. F. and Link, T. E.: Linked spatial variability of throughfall amount and intensity during rainfall in a coniferous forest, Agric. For. Meteorol., 248, 15–21, https://doi.org/10.1016/j.agrformet.2017.09.006, 2018.
Lal, R.: Soil conservation and ecosystem services, Int. Soil Water Conserv. Res., 2, 36–47, 2014.
Levia, D. F., Hudson, S. A., Llorens, P., and Nanko, K.: Throughfall drop size distributions: a review and prospectus for future research, WIREs Water, 4, e1225, https://doi.org/10.1002/wat2.1225, 2017.
Levia, D. F., Nanko, K., Amasaki, H., Giambelluca, T. W., Hotta, N., Iida, S., Mudd, R. G., Nullet, M. A., Sakai, N., Shinohara, Y., Sun, X., Suzuki, M., Tanaka, N., Tantasirin, C., and Yamada, K.: Throughfall partitioning by trees, Hydrol. Process, 33, 1698–1708, https://doi.org/10.1002/hyp.13432, 2019.
Liu, J., Liu, W., Zhang, W., and Wang, P.: The detected effects of driptips and leaf wettability on the kinetic energy of throughfall in rubber-based agroforestry in Xishuangbanna, Ecohydrology, 16, e2492, https://doi.org/10.1002/eco.2492, 2022.
Misik, T. and Kárász, I.: Low understory condition in an oak forest in Hungary, 1972 and 2022 – Síkfőkút Project is 50 years old, Acta Biologica Plantarum Agriensis, 10, 22–35, https://doi.org/10.21406/abpa.2022.10.22, 2022.
Miura, S., Yoshinaga, S., and Yamada, T.: Protective effect of floor cover against soil erosion on steep slopes forested with Chamaecyparis obtusa (hinoki) and other species, J. Forest Res., 8, 27–35, 2003.
Murata, I., Saruki, S., Kubota, K., Inoue, S., Tashiro, N., Enoki, T., Utsumi, and Y. Inoue, S.: Effects of sika deer (Cervus nippon) and dwarf bamboo (Sasamorpha borealis) on seedling emergence and survival in cool-temperate mixed forests in the Kyushu Mountains, J. Forest Res., 14, 296–301, 2009.
Nanko, K., Mizugaki, S., and Onda, Y.: Estimation of soil splash detachment rates on the forest floor of an unmanaged Japanese cypress plantation based on field measurements of throughfall drop sizes and velocities, Catena, 72, 348–361, 2008.
Nanko, K., Watanabe, A., Hotta, N., and Suzuki, M.: Physical interpretation of the difference in drop size distributions of leaf drips among tree species, Agric. For. Meteorol., 169, 74–84, https://doi.org/10.1016/j.agrformet.2012.09.018, 2013.
Nanko, K., Giambelluca, T. W., Sutherland, R. A., Mudd, R. G., Nullet, M. A., and Ziegler, A. D.: Erosion potential under Miconia calvescens stands on the island of Hawai'I, Land Degrad. Dev., 26, 218–226, 2015.
Nanko, K., Onda, Y., Kato, H., and Gomi, T.: Immediate change in throughfall spatial distribution and canopy water balance after heavy thinning in a dense mature, Ecohydrology, 9, 300–314, 2016.
Nanko, K., Tanaka, N., Leuchner, M., and Levia, D. F.: Throughfall Erosivity in Relation to Drop Size and Crown Position: A Case Study from a Teak Plantation in Thailand, Forest-Water Interactions, Ecological Studies. Springer International Publishing, Cham, 279–298, https://doi.org/10.1007/978-3-030-26086-6_12, 2020.
Nanko, K., Keim, R. F., Hudson, S. A., and Levia, D. F.: Throughfall drop sizes suggest canopy flowpaths vary by phenophase, J. Hydrol., 612, 128–144, 2022.
Pimentel, D. and Burgess, M.: Soil erosion threatens food production, Agriculture, 3, 443–463, 2013.
R Core Team: R: A Language And Environment for Statistical Computing, R Foundation for Statistical Computing, Vienna, Austria, https://www.R-project.org/ (last access: 2 December 2012), 2019.
Safari, A., Kavian, A., Parsakhoo, A., Saleh, I., and Jordán, A.: Impact of different parts of skid trails on runoff and soil erosion in the Hyrcanian forest (northern Iran), Geoderma, 263, 161–167, 2016.
Scholten, T., Geißler, C., Goc, J., Kühn, P., and Wiegand, C.: A new splash cup to measure the kinetic energy of rainfall, J. Plant. Nutr. Soil Sc., 174, 596–601, 2011.
Seitz, S., Goebes, P., Song, Z., Bruelheide, H., Härdtle, W., Kühn, P., Li, Y., and Scholten, T.: Tree species and functional traits but not species richness affect interrill erosion processes in young subtropical forests, Soil, 2, 49–61, 2016.
Seitz, S., Nebel, M., Goebes, P., Käppeler, K., Schmidt, K., Shi, X., Song, Z., Webber, C. L., Weber, B., and Scholten, T.: Bryophyte-dominated biological soil crusts mitigate soil erosion in an early successional Chinese subtropical forest, Biogeosciences, 14, 5775–5788, https://doi.org/10.5194/bg-14-5775-2017, 2017.
Shinohara, Y., Ichinose, K., Morimoto, M., Kubota, T., and Nanko, K.: Factors influencing the erosivity indices of raindrops in Japanese cypress plantations, Catena, 171, 54–61, 2018.
Song, Z., Seitz, S., Li, J., Goebes, P., Schmidt, K., Kühn, P., Shi, X., and Scholten, T.: Tree diversity reduced soil erosion by affecting tree canopy and biological soil crust development in a subtropical forest experiment, For. Ecol. Manag., 444, 69–77, 2019.
Takatsuki, S.: Effects of sika deer on vegetation in Japan: a review, Biol. Conserv., 142, 1922–1929, 2009.
van Dijk, A. I. J. M., Bruijnzeel, L. A., Rosewell, and C. J.: Rainfall intensitykinetic energy relationships: a critical literature appraisal, J. Hydrol., 261, 1–23, 2002.
Wang, P. K. and Pruppacher, H. R.: Acceleration to terminal velocity of cloud and raindrops, J. Appl. Meteorol. Climatol., 16, 275–280, 1977.
Yao, X., Yu, K., Wang, G., Deng, Y., Lai, Z., Chen, Y., Jiang, Y., and Liu, J.: Effects of soil erosion and reforestation on soil respiration, organic carbon and nitrogen stocks in an eroded area of Southern China, Sci. Total Environ., 683, 98–108, 2019.
Zemke, J. J.: Runoff and soil erosion assessment on forest roads using a small scale rainfall simulator, Hydrology, 3, 25, https://doi.org/10.3390/hydrology3030025, 2016.