the Creative Commons Attribution 4.0 License.
the Creative Commons Attribution 4.0 License.
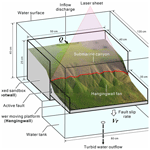
Evolution of submarine canyons and hanging-wall fans: insights from geomorphic experiments and morphodynamic models
Steven Y. J. Lai
David Amblas
Aaron Micallef
Hervé Capart
Tectonics play a significant role in shaping the morphology of submarine canyons, which form essential links in source-to-sink (S2S) systems. It is difficult, however, to investigate the resulting morphodynamics over the long term. For this purpose, we propose a novel experimental approach that can generate submarine canyons and hanging-wall fans on continuously evolving active faults. We utilize morphometric analysis and morphodynamic models to understand the response of these systems to fault slip rate (Vr) and inflow discharge (Q). Our research reveals several key findings. Firstly, the fault slip rate controls the merging speed of submarine canyons and hanging-wall fans, which in turn affects their quantity and spacing. Additionally, the long profile shapes of submarine canyons and hanging-wall fans can be decoupled into a gravity-dominated breaching process and an underflow-dominated diffusion process, which can be described using a constant-slope relationship and a morphodynamic diffusion model, respectively. Furthermore, both experimental and simulated submarine canyon–hanging-wall fan long profiles exhibit strong self-similarity, indicating that the long profiles are scale independent. The Hack's scaling relationship established through morphometric analyses serves as an important link between different scales in S2S systems, bridging laboratory-scale data to field-scale data and submarine-to-terrestrial relationships. Lastly, for deep-water sedimentary systems, we propose an empirical formula to estimate fan volume using canyon length, and the data from 26 worldwide S2S systems utilized for comparison show a strong agreement. Our geomorphic experiments provide a novel perspective for better understanding of the influence of tectonics on deep-water sedimentary processes. The scaling relationships and empirical formulas we have established aim to assist in estimating volume information that is difficult to obtain during long-term landscape evolution processes.
- Article
(21223 KB) - Full-text XML
-
Supplement
(3947 KB) - BibTeX
- EndNote
Global bathymetric data have been widely used to provide an overview of the distribution and geological significance of submarine canyons on active and passive margins (Harris and Whiteway, 2011; Harris et al., 2014). Source-to-sink (S2S) systems describe the response of the Earth's surface to tectonic and climatic signals over geological times, from terrestrial drainages (source) to deep-sea fans (sink) (Sømme et al., 2009; Nyberg et al., 2018). General scaling relationships between morphometric parameters and morphology have been established in the analyses of S2S systems (Sømme et al., 2009; Nyberg et al., 2018; Bernhardt and Schwanghart, 2021; Soutter et al., 2021b; Bührig et al., 2022a, b). Recent investigation of modern S2S systems with submarine-canyon configuration also highlight the significant influence of tectonics settings on canyon geomorphology (Soutter et al., 2021b; Bührig et al., 2022a, b). The identified scaling relationships provide insights into sedimentary controls and basin evolution and can even be used to predict relationships in systems lacking data (Sømme et al., 2009; Nyberg et al., 2018). The efficiency of sediment routing from land to the ocean depends on the position of submarine canyon heads with regards to terrestrial sediment sources. Bernhardt and Schwanghart (2021) find that steep and narrow shelves, as well as resistant bedrock and high river water discharge, facilitate shore-connected canyon occurrence. A recent study based on modern global bathymetric data identified potential predictors of canyon geomorphology and suggested that the relative magnitudes of canyon margin erosion and intra-canyon deposition are similar across different environmental settings (Bührig et al., 2022a). A similar meta-study examined the influence of tectonic settings on canyon geomorphology, revealing that although the tectonic setting exerts control on canyon geomorphology, the overall canyon geomorphology is not generally different across various tectonic scenarios (Bührig et al., 2022b). These findings enhance our understanding of slope systems and the role of tectonic setting in shaping deep-water sedimentary systems.
Other studies have explored the role of tectonics in shaping canyon morphology. For example, Covault et al. (2011) differentiated submarine canyon longitudinal profiles based on their convexity or concavity, revealing distinct depositional architectures corresponding to different continental-margin types. This study demonstrated that the shape of these profiles reflects the interplay between uplift, depositional relief construction and erosion related to mass wasting, providing a basis for classifying deep-sea sedimentary systems. Soutter et al. (2021b) re-examined the factors influencing the concavity of submarine canyons by analyzing the long profiles of 377 canyons. Their results indicated that tectonics is the primary control on canyon concavity, with active margins hosting the least concave profiles. Bourget et al. (2011) found that the Makran accretionary prism, between Pakistan and Iran, exhibits variability in tectonics and fluvial input distribution, which affects the turbidite system architecture and sediment distribution. Deep-water sedimentation on active margins involves complex sediment transport pathways, as highlighted by McArthur et al. (2022) in the Hikurangi subduction margin, where sediment input points and tortuous sediment dispersal corridors result in convoluted depositional systems, challenging simple models of basin fill.
Concerning the distal part of S2S systems, recent studies on submarine fans have developed scaling relationships to estimate fan volumes, while the submarine hanging-wall fans formed in syn-rift successions have distinct characteristics compared to traditional submarine fans. For instance, the study by Prélat et al. (2010) compared submarine lobes from six systems and identified two distinct populations related to basin floor topography. Despite differences in configurations and sediment supply, these lobes share similar characteristics. The study also concludes that basin floor topography influences lobe geometry, while channel avulsion influences lobe volumes. In a recent study, morphometric analysis of submarine fans revealed scaling relationships between channels and lobe-shaped bodies, providing insight into their architectural development (Pettinga et al., 2018). The study demonstrated that scaling relationships exist between channel dimensions and lobe-shaped body dimensions, allowing for the prediction of lobe body volume and depositional area. Unlike traditional submarine fans, submarine hanging-wall fans were identified in syn-rift successions. For instance, McArthur et al. (2013) investigated the stratigraphic development of an Upper Jurassic syn-rift succession in the Inner Moray Firth Basin in northeastern Scotland. The study showed that sedimentation rates varied throughout different phases of rifting, with overall rates comparable to other deep marine rift basins. Barrett et al. (2021) also demonstrated that the volume of footwall-sourced hanging-wall fans (Leeder and Gawthorpe, 1987) can be compared to the volume of material eroded from the fault scarp, revealing areas of sediment bypass and areas fed by sediment sources beyond the degraded fault scarp.
Compared to S2S studies and field surveys, only a few investigations have used numerical models or geomorphic experiments to study the long-term evolution of submarine canyons and fans. For example, a numerical surface process model was presented to examine submarine erosion processes caused by landslides and hyperpycnal flows (Petit et al., 2015). Their model demonstrated that the frequency of hyperpycnal flows largely influences the development of submarine canyons and that an increase in the submarine slope accelerated erosion and the formation of a more dendritic canyon network. Additionally, a hydraulic-based stratigraphic forward model was used to investigate the impacts of morphological parameters on sediment budget partitioning and the channel network of delta–canyon–fan systems on passive margins (Wan et al., 2022a, b). Their model demonstrated that submarine canyons retreat landward, tributaries develop on the outer banks of canyons and blind canyons expand landward. They concluded that the upslope pattern remains dominant regardless of changes in fluvial discharge and morphologies.
In terms of geomorphic experiments, pioneer microscale tank experiments were conducted to investigate the incision of a sediment bed by a gravity current (Métivier et al., 2005; Weill et al., 2014). The results indicated that the slope influences the erosion rate and channel incision speed, while brine discharge controls the channel geometry. Lai et al. (2016) emphasized the influence of tectonics on submarine canyon morphology and demonstrated that by isolating two key processes – the progressive growth of slope relief and a constant source of unconfined gravity flows – it is possible to produce a canyon growth sequence and morphologies that resemble those observed in the field. The study showed that unconfined gravity flows create featureless submarine slopes, whereas flows cascading across the shelf break result in deeply incised canyons with well-developed channel networks. Geomorphic experiments on self-channelized subaqueous fans revealed the formation, migration and abandonment of well-defined depocenters characterized by channels bounded by levees (Cantelli et al., 2011). The overall pattern of grain size variation is downstream fining, with sediment in the channels being coarser than in the levees. Similar experiments further demonstrated the crucial role of the break in slope in channel aggradation and lobe architecture (Fernandez et al., 2014). Geomorphic experiments were also conducted to study submarine fans formed by sediment-laden flows. Ferguson et al. (2020) found that depositional relief and compensational stacking led to markedly different deposits during waxing and waning phases. Soutter et al. (2021a) demonstrated that different types of topographic confinement affect turbidites and erosion, leading to variations in deposit thickness, bifurcation, onlap, lateral spreading, and plunge-pool formation.
Among these geomorphic experiments, very few studies have examined the long-term evolution of submarine canyons and fans under the joint of tectonics and gravity flows. Furthermore, there have been several metadata studies (Sømme et al., 2009; Nyberg et al., 2018; Bührig et al., 2022a, b) that have established relationships between various parameters in S2S systems using morphometric analysis. However, correlating sediment volumes to canyon data analysis remains very limited, and the challenge of quantifying volume changes in submarine canyons and fans over long-term evolutionary processes persists. To pursue this avenue, the present study improves the experimental method of Lai et al. (2016) and proposed a novel experimental approach to examine the ongoing development of submarine canyons and hanging-wall fans with density underflows on a continuously descending active fault. Through morphometric analysis and morphodynamic modeling, we aim to understand the response of submarine canyons and hanging-wall fans to fault slip rate (Vr) and inflow discharge (Q) and to establish cross-scale scaling relationships to facilitate the estimation of volumetric data during the evolutionary process, a challenging task in field studies. The objectives of this study include (1) establishing high-resolution digital elevation models (DEMs) through physical experiments; (2) developing laboratory-scale morphometric analysis and establishing scaling relationships between parameters; (3) comparing long profiles of canyons and hanging-wall fans between experiments and morphodynamic models; (4) identifying self-similarity within the system; and (5) proposing scaling relationships and empirical formulas across scales, extending from laboratory setup to the natural environment.
2.1 Experimental design
A novel experimental setup, containing a water tank (135 cm long, 80 cm wide and 75 cm deep) and a submerged sedimentary basin (75 cm long, 50 cm wide and 25 cm deep), was designed to investigate the evolution of submarine canyons and hanging-wall fans (Fig. 1). This submerged sedimentary basin consists of an upper fixed sandbox (as a footwall) and a lower moving platform (as a hanging-wall). The hanging wall was controlled by a motor with adjustable speed to simulate different fault slip rate (Vr) of 90° dip angle. Very fine silica sand (d50=0.1 mm) and kaolinite (proportion 100:1 by weight, as suggested by Hasbargen and Paola, 2000 and Lai et al., 2016) were well mixed and filled into the submerged sandbox and platform as an erodible substrate. Upstream, different inflows discharge (Q) of saturated brine (density ρin=1200 kg m−3) were used as unconfined downslope high-density turbidity currents for transporting sediment along submarine canyons and hanging-wall fans (Métivier et al., 2005; Spinewine et al., 2009; Sequeiros et al., 2010; Weill et al., 2014; Foreman et al., 2015; Lai et al., 2016, 2017). This approach contrasts with other experiments using dilute turbidity currents for forming classical submarine fans (Cantelli et al., 2011; Fernandez et al., 2014; Ferguson et al., 2020; Soutter et al., 2021a).
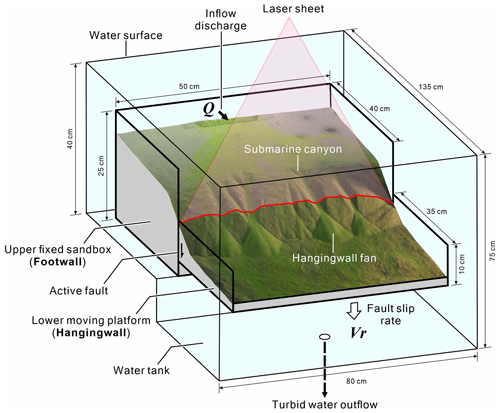
Figure 1The novel experimental setup for studying the evolution of submarine canyons and hanging-wall fans.
Six experimental runs were performed with different inflow discharges and fault slip rates (Table 1). In Series A, the inflow discharge (Q=1600 mm3 s−1) was constant, while Run A1 had a lower fault slip rate (Vr=0.025 mm s−1) and Run A2 had a faster fault slip rate (Vr=0.049 mm s−1). In Series B, the inflow discharge was doubled (Q∼3400 mm3 s−1), while Run B1 had a lower fault slip rate (Vr=0.026 mm s−1) and Run B2 had a faster fault slip rate (Vr=0.041 mm s−1). In Series C, extreme conditions were tested. For Run C1 the inflow discharge (Q=5000 mm3 s−1) was tripled and the fault slip rate was set to the slowest (Vr=0.014 mm s−1), while for Run C2 the inflow discharge (Q=800 mm3 s−1) was largely reduced and the fault slip rate was set to the fastest (Vr=0.064 mm s−1). Each experiment was divided into 7 to 12 successive stages; the stage interval was 10 min for the runs with relatively slow fault slip rate (i.e., Run A1, Run B1 and Run C1) and 5 min for the runs with relatively faster fault slip rate (i.e., Run A2, Run B2 and Run C2). Time-lapse photography was used to record the evolution of submarine canyon–fan systems every 5 s for each experiment. Without draining out the ambient water, the inflow was turned off to form a temporarily frozen landscape at the end of each stage. The newly generated submarine landscape was then scanned. A topographic imaging system (Lai et al., 2016, 2017; Huang et al., 2023) was used to construct high-resolution (1 mm × 1 mm) digital elevation models (DEMs), orthorectified images and gradient maps over successive stages.
2.2 Morphometric definitions of submarine canyons and hanging-wall fans
Morphological features of submarine canyons and hanging-wall fans were defined prior to applying the morphometric analysis (Fig. 2a). First, the fan apex was defined at the most upstream point of a fan, or the intersection between two fan edge asymptotes. Following this, canyon length (Lc) was defined as the maximum path from the canyon head to its fan apex, canyon width (Wc) was defined as the width of the bounding box for a canyon and canyon area (Ac) was defined the area of a canyon drainage. Similarly, fan length (Lf) was defined as the maximum path from the fan apex to its fan toe, fan width (Wf) was defined as the width of the bounding box for a fan and fan area (Af) was defined the area of a fan deposit. The volumes of submarine canyons and hanging-wall fans can be obtained by subtracting the pseudo-continental slope from the DEM of each stage (Fig. 2b). First, three long profiles were extracted from the DEM, which were unaffected by saline underflows (e.g., long profiles 1, 2 and 3 in Fig. 2a). The averaged long profiles were then used to create an averaged surface of continental slope for that stage (Fig. 2b). Next, the averaged surface of continental slope was subtracted from the DEM to obtain the DEM of difference (DoD). Negative values represent canyon incision depths, while positive values represent fan thicknesses.
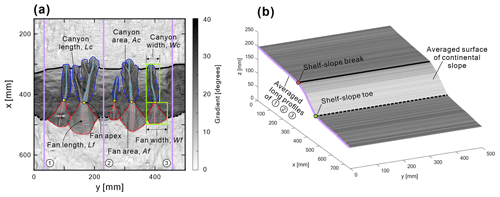
Figure 2(a) Morphometric definitions of submarine canyons and hanging-wall fans. Yellow dots are apexes of hanging-wall fans. Green rectangles are bounding boxes. The thick solid black line is the shelf–slope break. The thick dashed black line is shelf–slope toe. (b) Averaged surface of continental slope unaffected by saline underflows.
2.3 Geometric and morphodynamic models
The formation of submarine canyons and hanging-wall fans involve simultaneous erosion on the footwall and deposition on the hanging wall (Fig. 3), which resembles the fluvial process across an active fault (Hanks et al., 1984) and the knickpoint smoothing process observed in submarine canyons (Mitchell, 2006). Based on these geomorphic characteristics, we decoupled the formation into two different processes: (1) the evolution of a continental slope (Fig. 3b), which can be modeled by a constant-slope geometric model driven by a normal fault, and (2) the evolution of a submarine canyon and hanging-wall fan (Fig. 3c), which can be simulated by a simple morphodynamic model driven by an underflow.
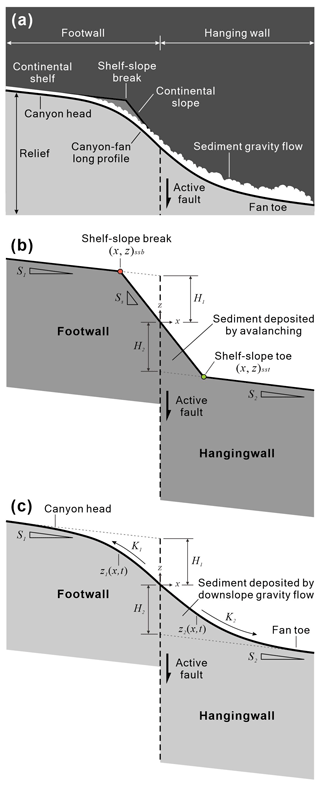
Figure 3(a) The formation of submarine canyons and hanging-wall fans. (b) Geometric definitions for the evolution of continental slope (modified from Lai et al., 2016). (c) Morphodynamic definitions for the evolution of submarine canyons and hanging-wall fans (modified from Lai and Wu, 2021).
For the continental slope (Fig. 3b), the position of the active fault was set to x=0. As the hanging wall continues to fall, the continental slope is formed by avalanching process, with its slope kept at the angle of repose. The retreating shelf–slope break and advancing shelf–slope toe can be described by the following geometric relationships (Eqs. 1 and 2) (modified from Lai et al., 2016):
where H1 and H2 are the upstream and downstream knickpoint heights and S1 and S2 are the upstream and downstream far field slopes, respectively. Ss is the inclination of continental slope.
For the submarine canyon and hanging-wall fan (Fig. 3c), the entire long profile can be described by a knickpoint smoothing process. Equations (3) and (4) describe the long profile of submarine canyon z1(x1,t) and the long profile of hanging-wall fan z2(x2,t), respectively:
where H is the knickpoint height (shape factor), erf is the error function (shape function), K is the diffusivity and S is the far-field slope. The first and second terms on the right-hand side of Eqs. (3) and (4) account for the variations in bed profile caused by the geomorphic diffusion and initial bed slope, respectively. The diffusion processes on footwall and hanging wall may be different, suggesting that different parameters (H1, K1, S1) and (H2, K2, S2) can be used for the submarine canyons and hanging-wall fans.
To investigate the self-similarity of the evolving canyon–fan long profiles in various settings, the first and second terms on the right-hand side of Eqs. (3) and (4) were normalized by the shape factor and the time-varying length scales H1 and and H2 and , respectively. Thus, the normalized profiles and varies in time only as a function of the dimensionless horizontal coordinate and (Capart et al., 2007; Lai and Wu, 2021):
The comparison between diffusion model and experiments is presented in Sect. 3.2.
3.1 Morphological evolution of submarine canyons and hanging-wall fans
The geomorphic experiments documented the initiation and complex development of submarine canyons and hanging-wall fans (Fig. 4). Taking Run B1 as an example, the fault location in the experiment was at x=400 mm (Fig. 4a). Once the experiment began, the hanging wall steadily descended at a rate of Vr=0.026 mm s−1, while the upstream released saline water with a discharge of Q=3300 mm3 s−1. As the hanging wall continued to descend, the shelf–slope break retreated upstream and the shelf–slope toe extended downstream (Fig. 4c). Along the slope where the saline underflow passed, a series of submarine canyons and hanging-wall fans formed. In areas without saline underflow, the slope maintained a fixed angle (approximately 38°, the angle of repose of the material). At t=30 min (Fig. 4d), four distinct canyon–hanging-wall fan systems (Systems A, B, C, and D) emerged on the continental slope. Additionally, slope-confined canyons occurred between Systems B and C (Fig. 4e), with their canyon heads located below the shelf–slope break. By t=70 min (Fig. 4h), these four canyon–hanging-wall fan systems continued to grow, but the original slope-confined System A vanished, while Systems B and C still maintained shelf-incising canyons. On the other hand, System D transformed into a slope-confined canyon. For detailed evolution processes of each run, please refer to Fig. S1–S6 and Videos S1–S6 in the Supplement.
Hill-shaded gradient maps demonstrated the details of submarine canyons and hanging-wall fans, as well as the similarities and dissimilarities between different runs (Fig. 5). In Series A (Fig. 5a and b), the inflow discharge was constant (Q=1600 mm3 s−1). The fault slip rate for Run A1 was Vr=0.025 mm s−1, while the fault slip rate for Run A2 was Vr=0.049 mm s−1. When Q was kept consistent, doubling the fault slip rate (Run A2, Fig. 5b) led to the rapid merge of canyons and hanging-wall fans into a few major systems, with significant increases in the length, width, and area of the systems. In Series B (Fig. 5c and d), the inflow discharge (Q≅3400 mm3 s−1) was twice as large as in Series A, but the fault slip rate remained similar to Series A (Vr=0.026 mm s−1 for Run B1, Vr=0.041 mm s−1 for Run B2). Similarly, when the fault slip rate doubled (Run B2, Fig. 5d), canyons and hanging-wall fans also rapidly merged into a few major systems, with significant increases in the length, width, and area of the systems. Lastly, Series C (Fig. 5e and f) presented two extreme cases. Run C1 demonstrated extremely high flow rate (Q=5000 mm3 s−1) paired with an extremely low fault slip rate (Vr=0.014 mm s−1), while Run C2 represented extremely low flow rate (Q=800 mm3 s−1) paired with an extremely high fault slip rate (Vr=0.064 mm s−1). Although the morphological differences in canyons and hanging-wall fans produced under these extreme conditions were quite significant, the same conclusion held: Vr controlled the overall morphological evolution. For example, at t=70 min, Run C1 still had seven canyon–hanging-wall fan systems (Fig. 5e), whereas Run C2 maintained almost a single major system throughout the entire evolution process (Fig. 5f), with larger length, width, and area compared to all systems in Run C1. In summary, fault slip rate controlled the merging speed of submarine canyons and hanging-wall fans, thereby influencing the quantity and spacing of the systems.
3.2 DEM analysis of canyon erosion and fan deposition
The DEM of differences (DoDs) shows the erosion depth of submarine canyons and the deposition thickness of hanging-wall fans (Fig. 6). For example, at t=30 min, runs with lower fault slip rates, such as Run A1, Run B1, and Run C1 (Fig. 6a–c, respectively), have shallower submarine canyon incision depths and hanging-wall fan deposition thicknesses. By contrast, runs with higher fault slip rates, such as Run A2, Run B2, and Run C2 (Fig. 6d–f, respectively), have deeper incision depths and deposition thicknesses. Additionally, since no sediment was added upstream in our experiments, the eroded sediment comes entirely from the substrate on the continental slope. The variations in the DoDs generated in the system are all related to changes in the bed load. Therefore, the volume of submarine canyons and their corresponding hanging-wall fans are similar.
The volume evolution of submarine canyon (Vc) demonstrates the influence of fault slip rate and inflow discharge on the system (Fig. 7). When the inflow discharge was fixed at Q=1600 mm3 s−1, the fault slip rate of Run A2 was twice as large as Run A1 (Fig. 7a). At a fixed time, Vc of Run A2 was 5 times larger than Run A1. Similarly, when Q=3400 mm3 s−1, the fault slip rate of Run B2 was twice as large as Run B1 (Fig. 7b), and the corresponding Vc followed the same trend. In contrast, when the fault slip rate was fixed at Vr=0.025 mm s−1, although the inflow discharge of Run B1 was twice as large as Run A1 (Fig. 7c), there was little difference in Vc between Run B1 and Run A1 over time. Similarly, when the fault slip rate was fixed at Vr=0.045 mm s−1 (Fig. 7d), the discharge of Run B2 was twice as large as Run A2, and the corresponding Vc was similar. This indicated that under fixed fault slip rate conditions, the magnitude of discharge did not cause significant variations in Vc. Finally, under extreme conditions, the slip rate of Run C2 was about 5 times larger than Run C1, although the flow rate of Run C2 was only Q=800 mm3 s−1 (the smallest among all runs), the corresponding Vc of Run C2 was 3 times larger than Run C1. All these results confirmed that fault slip rate had a more dominant influence on submarine canyons and hanging-wall fans when compared to inflow discharge.
3.3 Longitudinal profiles of submarine canyons and hanging-wall fans
The comparison between the experimental continental slopes and long profiles simulated by the geometric relationships (Eqs. 1 and 2) is shown in Fig. 8. The continental slope was extracted from an area unaffected by saline underflow, representing the morphological result solely generated by gravity in the system. Although the continental slope at the laboratory scale rested at the angle of repose (approximately 38°), this was the ultimate result of mass wasting processes, including landslides, flow sliding and gradual breaching. Despite the different fault slip rates given in different runs, the experimental continental slopes were consistent with the long profiles simulated by the geometric relationships. This indicates that the continental slope generated under different fault slip rates maintained a constant slope relationship at each stage.
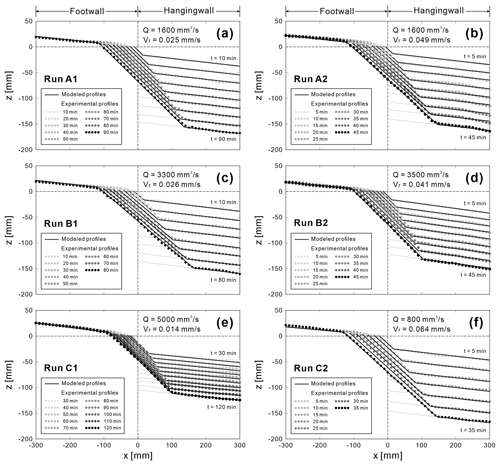
Figure 8Comparisons between the experimental and simulated continental slopes in the different runs.
The experimental long profiles of submarine canyons and hanging-wall fans were compared to the morphodynamic model (Fig. 9). Using System A in each experiment as an example, the results show that the morphodynamic model captured the long-term evolution trends of submarine canyons and hanging-wall fans. The diffusion coefficient K1 in the model controls the development of the canyon thalweg, while the diffusion coefficient K2 controls the development of the hanging-wall fan. As the hanging wall continues to descend, the canyon–hanging-wall fan long profile becomes smoother. This indicates that K1 and K2 are not fixed values but vary proportionally with the relief. Consequently, the morphodynamic model is validated and could be used to predict the long profile evolution of submarine canyons and hanging-wall fans at laboratory scale.
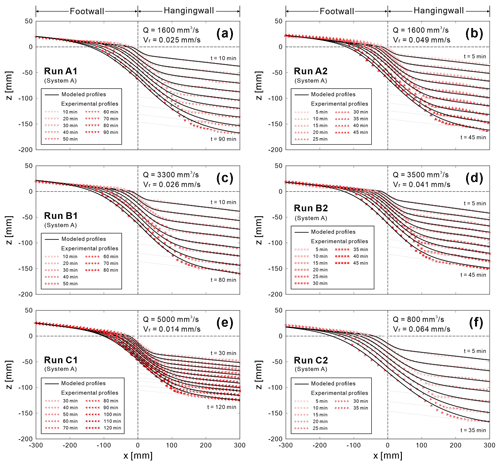
Figure 9Comparisons between experimental and simulated submarine canyon–hanging-wall fan long profiles in the different runs.
Finally, both experimental and simulated submarine canyon–hanging-wall fan long profiles plotted in dimensionless axes show strong self-similarity (Fig. 10). To investigate the self-similarity of the evolving long profiles during different stages of each run, the first and second terms on the right-hand side of Eq. (3) are, respectively, normalized by the shape factor H and the time-varying length scale . That means that the upstream and downstream profiles, and , are plotted against the dimensionless coordinates σ1 and σ2. For each run, the scaled profiles of an evolving submarine canyon–hanging-wall fan collapse to a single diffusion-based theoretical profile (black solid line), indicating the establishment of morphological self-similarity consistently.
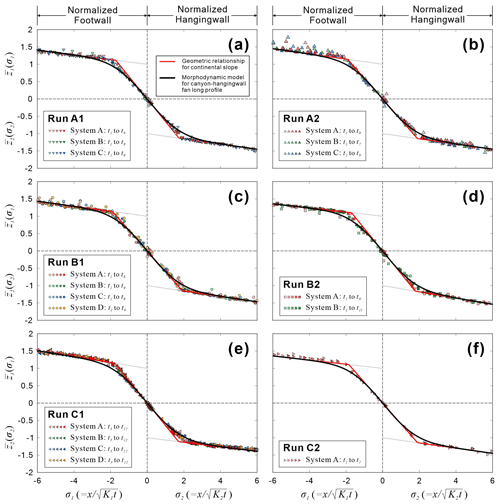
Figure 10Dimensionless long profiles for each run. Solid black lines are modeled dimensionless canyon–fan long profiles by two-diffusion theory. Solid red lines represent modeled dimensionless continental slopes. Solid gray lines show the normalized step function. Note that the numbers and times of each traced system are not identical.
3.4 Scaling relationships of submarine canyons and hanging-wall fans
The results of the morphometric analysis demonstrated that parameters in submarine canyons and hanging-wall fans exhibit strong scaling relationships (Fig. 11). For instance, there is a significant linear correlation between canyon length (Lc) and canyon area (Ac), which aligns with Hack's empirical relationship (Fig. 11a). The Hack's coefficient is 1.75, and the exponent coefficient is 0.51. In hanging-wall fans, we also observe a similar scaling relationship according to Hack's law between fan length (Lf) and fan area (Af) (Fig. 11b). The Hack's coefficient is 1.3, and the exponent coefficient remains 0.51 as well. Moreover, combining the canyon relief height (H1) and fan relief height (H2), as determined by the morphodynamic model in Sect. 3.3, we propose empirical formulas for estimating the canyon volume (Vec) and the fan volume (Vef) using the area and relief height. These formulas are as follows: Ve, where α represents the experimentally calibrated coefficient (α=0.1 in this study), and Ve, where β represents the experimentally calibrated coefficient (β=0.1 in this study). The results demonstrate a close correspondence between the estimated canyon volume (Vec) and fan volume (Vef) using our proposed empirical formulas and the directly measured canyon volume (Vc) and fan volume (Vf) obtained from DoDs (Fig. 11c and d).
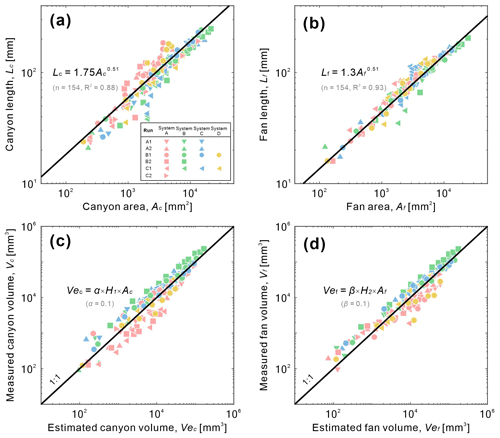
Figure 11(a, b) The scaling relationships of length to area established for submarine canyons and fans, respectively. (c, d) One-to-one relationship for the estimated to measured volumes of canyons and fans, respectively.
To validate the scaling relationship between length and area obtained from laboratory scale, we compared the laboratory data with field data (Fig. 12). The obtained results show that the length-to-area relationship obtained from laboratory-scale submarine canyons and hanging-wall fans is consistent with the field-scale submarine canyon length to fluvial drainage area analyzed in S2S studies (n=9477, Harris et al., 2014). Our data also aligns with modern metadata of submarine canyons configuration in active margins (n=35) and passive margins (n=36) (Bührig et al., 2022a, their Fig. 8). Additionally, we find that this relationship (L=1.75A0.51) also correlates well with the channel length to drainage area obtained from classic large fluvial rivers. For example, this length-to-area relationship is maintained in the Tennessee Valley (n=63, Montgomery and Dietrich, 1989), in a linear mountain belt (n=193, Hovius, 1996), in fault blocks (n=51, Talling et al., 1997), in the 50 largest rivers (n=50, Vörösmarty et al., 2000), in Death Valley (n=18, Bull, 1962; Denny, 1965) and even on Mars (Kraal et al., 2008). Moreover, the Hack's exponent falls within a reasonable range (0.46–0.56), e.g., the Hack's exponent is 1 for linear canyons and 0.5 for dendritic canyons of the Atlantic USA continental slope (Mitchell, 2005), the Hack's exponent is 0.46 for canyons in the south Ebro Margin (Micallef et al., 2014), and the Hack's exponent is 0.49 for terrestrial river drainage basins (Montgomery and Dietrich, 1992). Interestingly, our submarine canyons and hanging-wall fans show a striking similarity in scale to the experiments of coastal overwash morphology (n=96, Lazarus, 2016), defined as the sedimentary deposit of microtidal barrier, as well as field-scale overwash (Hudock lobes, n=117, Hudock et al., 2014; Core Bank, n=65, Lazarus, 2016), all exhibiting the same scaling relationship. Therefore, the Hack's scaling relationship established from our evolving submarine canyons and hanging-wall fans at the laboratory scale, spanning 22 orders and totaling 10 784 data points, serves as an important link across submarine to terrestrial domains and laboratory-scale to field-scale domains.
The main purpose of this study is to understand the response of submarine canyons and hanging-wall fans to fault slip rate and inflow discharge through geomorphic experiments and morphodynamic models. We aim to establish scaling relationships across scales to help estimate volume information through canyon–fan evolution, which is difficult to obtain through classical geomorphic and stratigraphic studies. Below, we address three key issues that arise from our research.
4.1 Why do geomorphic experiments work?
Geomorphic experiments are valuable tools for studying the processes and dynamics of landforms and landscapes. They involve manipulating physical variables in controlled environments to simulate natural conditions and understand their underlying mechanisms. By conducting these experiments, researchers can observe and measure how landforms and landscapes respond to factors like erosion, sediment transport, and deposition. These experiments provide valuable insights into the complex interactions between hydraulic, geomorphic, and sedimentological processes. Ultimately, researchers aim to identify self-similar laws or cross-scale relationships that exist within the system. In many cases, such experiments have proven feasible even for phenomena so far beyond the reach of numerical simulations.
For instance, in our experiments, we observed unprecedented evolution of submarine canyons and hanging-wall fans, including the merging phenomenon between canyons and the coalescing process of fans (Figs. 4, 5 and S1–S6), and the formation of drainage networks in submarine canyons (Video S1 to Video S6). These are long-term landscape evolution features that cannot be observed in the field. In addition, we found that the long profiles extracted from our experiments exhibit a high degree of self-similarity (Fig. 10), indicating that they are scale versions of each other. Furthermore, in our morphometric analyses, we demonstrated that Hack's scaling relationship is an empirical formula applicable across laboratory to field scales from submarine to terrestrial systems (Fig. 12). These results all support the scale independence and applicability of our experimental results.
In our experiment, all morphologies occurring on the continental slope originate from fault-slip-generated increasing relief. Submarine canyons and hanging-wall fans only appear in areas where density underflows flow through. In regions without fault movement, such as the continental shelf in the footwall region (Fig. 4b), there will be no morphological changes; i.e., underflows will bypass the continental shelf until they encounter the shelf–slope break, where morphological changes will start to occur. This is one of the key elements in our experimental approach. This study aligns with the conclusion of Lai et al. (2016) that the sustained formation of submarine canyons in the laboratory requires both increased relief and the presence of high-density underflows. We decouple this complex phenomenon into two main mechanisms. The first being breaching processes driven by gravity, including debris flows and mass wasting processes. At a laboratory scale, the morphological response is that the slope will maintain an angle of repose. The second breaching process involves submarine canyons and hanging-wall fans formed by saline underflow incision, transport and deposition. The underlying mechanisms we interpret are similar to the appearance of submarine hanging-wall fans in syn-rift successions (McArthur et al., 2013; Barrett et al., 2021), which involves mixing of turbidity currents and mass-wasting processes. We also agree that the volume of footwall-sourced hanging-wall fans are comparable to the volume of material eroded from the fault scarp (Barrett et al., 2021).
However, there is a notable scarcity of geomorphic experiments focused on submarine canyon evolution. Our experimental approach diverges from that of Métivier et al. (2005) and Weill et al. (2014). Their experimental submarine canyons started from a given slope-to-plain condition, and they measured the evolution of submarine gullies and lobes at the slope break. These studies do not emphasize the potential influence of continuous active tectonics on the evolution of submarine canyons. In addition, our experiments are different from those studies of submarine fan evolution (Cantelli et al., 2011; Fernandez et al., 2014; Ferguson et al., 2020), which were particularly designed to understand the sedimentary processes and stratigraphy formed by a point-source turbidity current on a ramp of constant slope. These experiments neither emphasize the role of tectonics that may influence the evolution of submarine canyons and submarine fans. Our approach to generating evolving dynamic continental slopes is extended from Lai et al. (2016). They achieved the continuous effect of tectonics (i.e., increasing relief) by continuously lowering the base level to provide sufficient substrate for underflows to erode, thereby forming submarine canyon drainages that can evolve over time. This approach is similar to the subaerial experiments conducted by Hasbargen and Paola (2000) for simulating the evolution of drainage systems through rainfall erosion caused by base level fall. It is also similar to the subaerial experiments conducted by Strak et al. (2011) on normal faults, which resulted in the formation of valleys and alluvial fans. In addition, mountain building experiments generated by uplifting and rainfall (Bonnet and Crave, 2003, 2006; Babault et al., 2005) also support the hypothesis that controlling increasing relief is the key to generate dynamic drainage systems over time. Our experimental approach can be seen as an extension of fluvial drainage experiments, applied to subaqueous environments with a focus on tectonics and underflow-driven deep-water sedimentary systems. Therefore, our current experimental approach for studying submarine canyons and hanging-wall fans is unique.
To conclude, we reaffirm the findings of our prior research demonstrating that both increasing relief and sediment gravity flows are the two foundation factors for the progressive development of submarine canyons at laboratory scale. We also underscore the scale-independence and self-similarity of submarine canyon–hanging-wall fan long profiles within our geomorphic experiments. Our experimental approach echoes the advantages of geomorphic experiments mentioned in Paola et al. (2009) and Lajeunesse et al. (2010), which have been demonstrated to be valuable in validating numerical models and aiding in the interpretation of field cases. However, readers must exercise caution when interpreting field observations in light of our experimental results. Our experiments only consider fault slip rate and inflow discharge, which creates highly simplified conditions. Additionally, factors such as grain size, different tectonic processes, turbidity currents with fine suspended deposits, water salinity, temperature, ocean currents and other environmental factors may lead to experimental results may diverge from real-world field scenarios.
4.2 Fault slip rate and inflow discharge control the morphology of submarine canyons and hanging-wall fans
We found that fault slip rate (Vr) has a greater control over the morphologies of submarine canyons and hanging-wall fans compared to inflow discharge (Q), especially affecting the number and spacing of systems, as well as the volume of erosion and deposition. When the fault slip rate is higher, retrograding (or breaching) processes on the continental slope and canyon walls become more intense. Under the same flow rate, saline underflows need to converge in order to maintain canyon entrenchment on the continental slope, facilitating the piracy of contiguous canyons. On the contrary, when the fault slip rate is lower, even under high flow rates, the excess flow will bypass the slope, resulting in insignificant effects on the morphology and depth changes of submarine canyons. For example, in Run A2 (Fig. 6d), Run B2 (Fig. 6e) and Run C2 (Fig. 6f), a higher fault slip rate will result in a decrease in the number of submarine canyons and hanging-wall fans, as well as a closer spacing between canyons. However, it will significantly increase the erosion volume of the submarine canyons (Fig. 7f). Our experimental results agree with the conclusion of Soutter et al., (2021b). The authors examined the factors controlling the concavity of submarine canyons by analyzing 377 modern canyons. Their results indicate that tectonics (similar to our fault slip rate condition) is the primary factor influencing concavity, with active margins having the least concave profiles. The position of the canyon and onshore climate (similar to our inflow discharge condition) also contribute but to a lesser extent. In summary, our experimental results indicate that the fault slip rate (i.e., tectonic effects) have a greater control over the morphologies of submarine canyons and hanging-wall fans compared to the inflow discharge (i.e., surface processes). Our conclusion is consistent with the conclusion of Soutter et al. (2021b); i.e., tectonics is the overriding control for deep-water sedimentary systems.
4.3 Submarine canyon morphometrics to estimate the volume of submarine fans
In Sect. 3.4, we revealed that Hack's scaling relationship (L=1.75A0.51) can link the length-to-area relationship of both subaerial and subaqueous examples, with the area values spanning across 22 orders of magnitude. Additionally, we also found in our experiments that canyon volumes can be estimated using canyon relief height and canyon area (Fig. 11c) and that fan volumes can be estimated using fan relief height and fan area (Fig. 11d). Therefore, we propose an empirical formula for estimating fan volume (Vf) in deep-water sedimentary systems using only the length of the submarine canyon (Lc). The formula is . This formula has been validated through our experiments and morphodynamic model (Fig. 13a) and does not require additional information from terrestrial drainages.
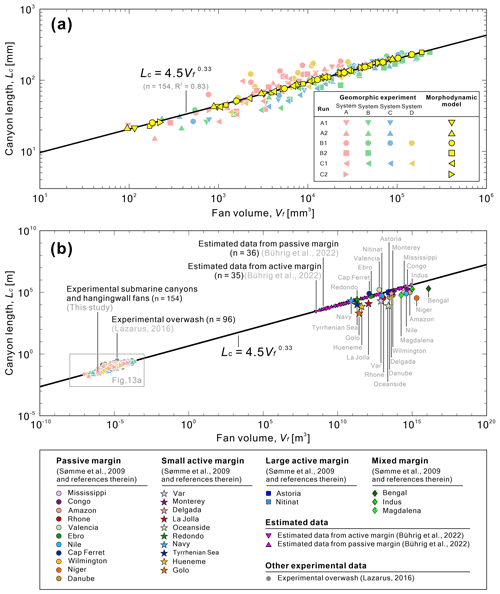
Figure 13(a) The scaling relationship built from our experimental data and verified by the morphodynamic model. (b) The scaling relationship of canyon lengths to fan volumes.
To validate this formula, we compared 26 field cases from global databases that have both canyon length and fan volume information (Sømme et al., 2009, and references therein). These cases include 11 examples from passive margins, 10 examples from small active margins, 2 examples from large active margins and 3 examples from mixed margins. We found that the estimated fan volumes using this formula align well with the observed data from these field cases (Fig. 13b). In addition, we estimated the corresponding fan volumes using 35 modern canyon length data from active margins and 36 modern canyon length data from passive margins (Bührig et al., 2022a, b). The estimated results align with the fan volumes documented in past global datasets (Sømme et al., 2009 and references therein). However, further field data is required to validate the accuracy of these estimations. To conclude, alongside Hack's scaling relationship, we propose a new empirical formula for estimating fan volumes using canyon lengths. We anticipate that this formula will aid in estimating sediment volumes in deep-water sedimentary systems at their terminus. However, it is important to approach the interpretation of this simple empirical formula with caution. For instance, is may not be suitable for estimating the lobe elements generated in the distal part of a submarine channel (Prélat et al., 2010; Pettinga et al., 2018).
In this paper, we propose a novel experimental approach to investigate the long-term geomorphic evolution processes of submarine canyons and hanging-wall fans that are simultaneously influenced by tectonics and surface flows. Through physical experiments, morphometric analysis, and morphodynamic modeling, we have gained insights into the response of submarine canyons and hanging-wall fans to fault slip rate (Vr) and inflow discharge (Q). We further presented scaling relationships that span from laboratory to field scales and from submarine canyons to terrestrial drainages. The following points are our major findings.
-
The experimental results show that fault slip rate controls the merging speed of submarine canyons and hanging-wall fans, thereby affecting their quantity and spacing. Compared to inflow discharge, fault slip rate has a more dominant influence on submarine canyons and hanging-wall fans and directly affects their volume. This conclusion is consistent with the overriding control of tectonics concluded from recent works on natural systems (e.g., Bernhardt and Schwanghart, 2021; Bührig et al., 2022a, b; McArthur et al., 2022; Soutter et al., 2021b).
-
The long profile shapes of submarine canyons and hanging-wall fans can be decoupled into gravity-dominated breaching processes and underflow-dominated diffusion processes, which can be described by a constant-slope relationship and a morphodynamic diffusion model, respectively.
-
The comparison between the experimental long profiles and our proposed constant-slope relationship and morphodynamic model shows a good agreement. Moreover, the long profiles of submarine canyons and hanging-wall fans exhibit strong self-similarity, indicating their scale independence.
-
The Hack's scaling relationship established through morphometric analyses is a robust relationship spanning 22 orders of magnitude and over 10 000 data points. This relationship is built upon laboratory-scale data to field-scale data and serves as an important link between different scales in source-to-sink systems.
-
For deep-water sedimentary systems, we propose an empirical formula to estimate fan volume based on canyon length. This formula shows good agreement with the comparison results from 26 representative source-to-sink systems worldwide. We also estimate fan volumes for recent data from active and passive margins (Bührig et al., 2022a, b), which fall within a reasonable range compared to the globally representative fan volumes in source-to-sink systems.
However, we do not claim that the submarine canyons and hanging-wall fans in our experiments are precise dynamical models of their field counterparts. Field examples result from complex, varied tectonic processes and underflow conditions, which may lead to significant discrepancies between the experiments and field cases. Therefore, when using our empirical formulas for interpretations, careful consideration of various tectonics and flow conditions for the specific field case is necessary. In addition, in the analysis of morphometric analysis, we did obtain data on canyon width and fan width. However, we have not yet discovered any interesting trends that can be compared to published data or help us predict hard-to-obtain volume information. Future research can continue to analyze these width data and perhaps establish more valuable relationships. In summary, our physical experiments provide a novel perspective to examine the long-term evolution processes of submarine canyons and hanging-wall fans. The proposed empirical formula may help field researchers estimate volume information from incomplete bathymetric and stratigraphic data.
Data are available at https://doi.org/10.5281/zenodo.7271139 (Lai, 2022).
The supplement related to this article is available online at: https://doi.org/10.5194/esurf-12-621-2024-supplement.
SYJL conceived the idea, performed the experiment and conducted the analysis. SYJL drafted the paper, with contributions from DA, AM and HC. All authors worked on the submitted final version.
The contact author has declared that none of the authors has any competing interests.
Publisher's note: Copernicus Publications remains neutral with regard to jurisdictional claims made in the text, published maps, institutional affiliations, or any other geographical representation in this paper. While Copernicus Publications makes every effort to include appropriate place names, the final responsibility lies with the authors.
This study has been supported by the Ministry of Science and Technology (MOST), Taiwan (grant no. MOST 109-2628-E-006-006-MY3), and the National Science and Technology Council (NSTC), Taiwan (grant no. NSTC 112-2628-E-006-009-MY3). Students of the Morphohydraulics Imaging Laboratory (MIL), Jyun-Fong Jiang, Sam Yan Jyun Huang, and Te-Min Kong, provided valuable assistance during the performance the experiments. David Amblas acknowledges the support from the Spanish government through grant no. PID2020-114322RBI00 funded by MCIN/AEI/10.13039/501100011033 and from the Catalan Government Excellence Research Groups (grant no. 2021-SGR-01195). Thomas P. Gerber and Peter T. Harris are acknowledged for providing early discussions about this research and global bathymetric data of submarine canyons, respectively.
This study has been supported by the National Science and Technology Council (NSTC), Taiwan (grant no. NSTC 112-2628-E-006-009-MY3).
This paper was edited by Tom Coulthard and reviewed by Adam Daniel McArthur, Morelia Urlaub, and Laura Henrika Bührig.
Babault, J., Bonnet, S., Crave, A., and Van Den Driessche, J.: Influence of piedmont sedimentation on erosion dynamics of an uplifting landscape: An experimental approach, Geology, 33, 301, https://doi.org/10.1130/g21095.1, 2005.
Barrett, B. J., Hodgson, D. M., Jackson, C. A. L., Lloyd, C., Casagrande, J., and Collier, R. E. L.: Quantitative analysis of a footwall-scarp degradation complex and syn-rift stratigraphic architecture, Exmouth Plateau, NW Shelf, offshore Australia, Basin Res., 33, 1135–1169, https://doi.org/10.1111/bre.12508, 2021.
Bernhardt, A. and Schwanghart, W.: Where and why do submarine canyons remain connected to the shore during sea-level rise? Insights from global topographic analysis and Bayesian regression, Geophys. Res. Lett., 48, e2020GL092234, https://doi.org/10.1029/2020GL092234, 2021.
Bonnet, S. and Crave, A.: Landscape response to climate change: Insights from experimental modeling and implications for tectonic versus climatic uplift of topography, Geology, 31, 123-126, https://doi.org/10.1130/0091-7613(2003)031<0123:LRTCCI>2.0.CO;2, 2003.
Bonnet, S. and Crave, A.: Macroscale dynamics of experimental landscapes, analogue and numerical modeling of crustal-scale processes, Special Publications, Geological Society, London, https://doi.org/10.1144/GSL.SP.2006.253.01.17, 2006.
Bourget, J., Zaragosi, S., Ellouz-Zimmermann, N., Mouchot, N., Garlan, T., Schneider, J.-L., Lanfumey, V., and Lallemant, S.: Turbidite system architecture and sedimentary processes along topographically complex slopes: the Makran convergent margin, Sedimentology, 58, 376-406, https://doi.org/10.1111/j.1365-3091.2010.01168.x, 2011.
Bührig, L. H., Colombera, L., Patacci, M., Mountney, N. P., and McCaffrey, W. D.: Tectonic influence on the geomorphology of submarine canyons: implications for deep-water sedimentary systems, Source or Sink? Erosional and Depositional Signatures of Tectonic Activity in Deep-Sea Sedimentary Systems, Front. Earth Sci., 10, 836823, https://doi.org/10.3389/feart.2022.836823, 2022a.
Bührig, L. H., Colombera, L., Patacci, M., Mountney, N. P., and McCaffrey, W. D.: A global analysis of controls on submarine-canyon geomorphology, Earth-Sci. Rev., 104150, https://doi.org/10.1016/j.earscirev.2022.104150, 2022b.
Bull, W. B.: Relations of alluvial fan size and slope to drainage basin size and lithology in western Fresno County, California, US Geological Survey Professional Paper 450, US Geological Survey, 51–53, https://books.google.com.tw/books?hl=zh-TW&lr=&id=HQbs8uBocCMC&oi=fnd&pg=PA51&dq=Bull,+W.+B.+(1962),+Relations+of+alluvial-fan+size+and+slope+to+drainage-basin+size+and+lithology+in+western+Fresno+County,+California+U.S.+Geol.+Surv.+Prof.+Pap.+450-B.&ots=iDArpjipLb&sig=1rffBDshQHMfhgvFQZuOAYHSGS0&redir_esc=y#v=onepage&q&f=false (last access: 1 November 2022), 1962.
Cantelli, A., Pirmez, C., Johnson, S., and Parker, G.: Morphodynamic and stratigraphic evolution of self-channelized subaqueous fans emplaced by turbidity currents, J. Sediment. Res., 81, 233-247, https://doi.org/10.2110/jsr.2011.20, 2011.
Capart, H., Bellal, M., and Young, D. L.: Self-similar evolution of semi-infinite alluvial channels with moving boundaries, J. Sediment. Res., 77, 13–22, https://doi.org/10.2110/jsr.2007.009, 2007.
Covault, J. A., Fildani, A., Romans, B. W., and McHargue, T.: The natural range of submarine canyon-and-channel longitudinal profiles, Geosphere, 7, 313–332, https://doi.org/10.1130/GES00610.1, 2011.
Denny, C. S.: Alluvial fans in the Death Valley region, California and Nevada, US Government Printing Office, 466 pp., https://pubs.usgs.gov/pp/0466/report.pdf (last access: 1 November 2022), 1965.
Fernandez, R. L., Cantelli, A., Pirmez, C., Sequeiros, O., and Parker, G.: Growth patterns of subaqueous depositional channel lobe systems developed over a basement with a downdip break in slope: Laboratory experiments, J. Sediment. Res., 84, 168-182, https://doi.org/10.2110/jsr.2014.10, 2014.
Foreman, B. Z., Lai, S. Y. J., Komatsu, Y., and Paola, C.: Braiding of submarine channels controlled by aspect ratio similar to rivers, Nat. Geosci., 8, 700–703, https://doi.org/10.1038/ngeo2505, 2015.
Ferguson, R. A., Kane, I. A., Eggenhuisen, J. T., Pohl, F., Tilston, M., Spychala, Y. T., and Brunt, R. L.: Entangled external and internal controls on submarine fan evolution: an experimental perspective, Depos. Rec., 6, 605–624, https://doi.org/10.1002/dep2.109, 2020.
Hanks, T. C., Bucknam, R. C., Lajoie, K. R., and Wallace, R. E.: Modification of wave-cut and faulting-controlled landforms, J. Geophys. Res.-Solid, 89, 5771–5790, https://doi.org/10.1029/JB089iB07p05771, 1984.
Harris, P. T. and Whiteway, T.: Global distribution of large submarine canyons: geomorphic differences between active and passive continental margins, Mar. Geol., 285, 69–86, https://doi.org/10.1016/j.margeo.2011.05.008, 2011.
Harris, P. T., Macmillan-Lawler, M., Rupp, J., and Baker, E. K.: Geomorphology of the oceans, Mar. Geol., 352, 4–24, https://doi.org/10.1016/j.margeo.2014.01.011, 2014.
Hasbargen, L. E. and Paola, C.: Landscape instability in an experimental drainage basin, Geology, 28, 1067–1070, https://doi.org/10.1130/0091-7613(2000)28<1067:LIIAED>2.0.CO;2, 2000.
Hovius, N.: Regular spacing of drainage outlets from linear mountain belts, Basin Res., 8, 29-44, https://doi.org/10.1111/j.1365-2117.1996.tb00113.x, 1996.
Huang, S. Y., Lai, S. Y., Limaye, A. B., Foreman, B. Z., and Paola, C.: Confinement width and inflow-to-sediment discharge ratio control the morphology and braiding intensity of submarine channels: insights from physical experiments and reduced-complexity models, Earth Surf. Dynam., 11, 615–632, https://doi.org/10.5194/esurf-11-615-2023, 2023.
Hudock, J. W., Flaig, P. P., and Wood, L. J.: Washover fans: A modern geomorphologic analysis and proposed classification scheme to improve reservoir models, J. Sediment. Res., 84, 854–865, https://doi.org/10.2110/jsr.2014.64, 2014.
Kraal, E. R., Asphaug, E., Moore, J. M., Howard, A., and Bredt, A.: Catalogue of large alluvial fans in martian impact craters, Icarus, 194, 101–110, https://doi.org/10.1016/j.icarus.2007.09.028, 2008.
Lai, S. Y., Hung, S. S., Foreman, B. Z., Limaye, A. B., Grimaud, J. L., and Paola, C.: Stream power controls the braiding intensity of submarine channels similarly to rivers, Geophys. Res. Lett., 44, 5062–5070, https://doi.org/10.1002/2017GL072964, 2017.
Lai, S. Y. J.: Experiments of submarine canyon-fan systems in fault-controlled margins, Zenodo [data set], https://doi.org/10.5281/zenodo.7271139, 2022.
Lai, S. Y. J. and Wu, F. C.: Two-stage transition from Gilbert to hyperpycnal delta in reservoir, Geophys. Res. Lett., 48, e2021GL093661, https://doi.org/10.1029/2021GL093661, 2021.
Lai, S. Y. J., Gerber, T. P., and Amblas, D.: An experimental approach to submarine canyon evolution, Geophys. Res. Lett., 43, 2741–2747, https://doi.org/10.1002/2015GL067376, 2016.
Lajeunesse, E., Malverti, L., Lancien, P., Armstrong, L., Metivier, F., Coleman, S., Smith, C. E., Davies, T., Cantelli, A., and Parker, G.: Fluvial and submarine morphodynamics of laminar and near-laminar flows: a synthesis, Sedimentology, 57, 1–26, https://doi.org/10.1111/j.1365-3091.2009.01109.x, 2010.
Lazarus, E. D.: Scaling laws for coastal overwash morphology, Geophys. Res. Lett., 43, 12113–12119, https://doi.org/10.1002/2016GL071213, 2016.
Leeder, M. and Gawthorpe, R.: Sedimentary models for extensional tilt-block/half-graben basins, Geol. Soc. Lond. Spec. Publ., 28, 139–152, 1987.
McArthur, A. D., Hartley, A. J., and Jolley, D. W.: Stratigraphic development of an Upper Jurassic deep marine syn-rift succession, Inner Moray Firth Basin, Scotland, Basin Res., 25, 285–309, https://doi.org/10.1111/j.1365-2117.2012.00557.x, 2013.
McArthur, A. D., Crisóstomo-Figueroa, A., Wunderlich, A., Karvelas, A., and McCaffrey, W. D.: Sedimentation on structurally complex slopes: neogene to recent deep-water sedimentation patterns across the central Hikurangi subduction margin, New Zealand, Basin Res., 34, 1807–1837, https://doi.org/10.1111/bre.12686, 2022.
Métivier, F., Lajeunesse, E., and Cacas, M.-C.: Submarine canyons in the bathtub, J. Sediment. Res., 75, 6–11, https://doi.org/10.2110/jsr.2005.002, 2005.
Micallef, A., Ribó, M., Canals, M., Puig, P., Lastras, G., and Tubau, X.: Space-for-time substitution and the evolution of a submarine canyon–channel system in a passive progradational margin, Geomorphology, 221, 34–50, https://doi.org/10.1016/j.geomorph.2014.06.008, 2014.
Mitchell, N. C.: Interpreting long-profiles of canyons in the USA Atlantic continental slope, Mar. Geol., 214, 75–99, https://doi.org/10.1016/j.margeo.2004.09.005, 2005.
Mitchell, N. C.: Morphologies of knickpoints in submarine canyons, Geol. Soc. Am. Bull., 118, 589–605, https://doi.org/10.1130/B25772.1, 2006.
Montgomery, D. R. and Dietrich, W. E.: Source areas, drainage density, and channel initiation, Water Resour. Res., 25, 1907–1918, https://doi.org/10.1029/WR025i008p01907, 1989.
Montgomery, D. R. and Dietrich, W. E.: Channel initiation and the problem of landscape scale, Science, 255, 826–830, https://doi.org/10.1126/science.255.5046.826, 1992.
Nyberg, B., Helland-Hansen, W., Gawthorpe, R. L., Sandbakken, P., Eide, C. H., Sømme, T., Hadler-Jacobsen, F., and Leiknes, S.: Revisiting morphological relationships of modern source-to-sink segments as a first-order approach to scale ancient sedimentary systems, Sediment. Geol., 373, 111–133, https://doi.org/10.1016/j.sedgeo.2018.06.007, 2018.
Paola, C., Straub, K., Mohrig, D., and Reinhardt, L.: The “unreasonable effectiveness” of stratigraphic and geomorphic experiments, Earth-Sci. Rev., 97, 1–43, https://doi.org/10.1016/j.earscirev.2009.05.003, 2009.
Petit, C., Migeon, S., and Coste, M.: Numerical models of continental and submarine erosion: application to the northern Ligurian Margin (Southern Alps, France/Italy), Earth Surf. Proc. Land., 40, 681–695, https://doi.org/10.1002/esp.3685, 2015.
Pettinga, L., Jobe, Z., Shumaker, L., and Howes, N.: Morphometric scaling relationships in submarine channel–lobe systems, Geology, 46, 819–822, https://doi.org/10.1130/G45142.1, 2018.
Prélat, A., Covault, J. A., Hodgson, D. M., Fildani, A., and Flint, S. S.: Intrinsic controls on the range of volumes, morphologies, and dimensions of submarine lobes, Sediment. Geol., 232, 66–76, https://doi.org/10.1016/j.sedgeo.2010.09.010, 2010.
Sequeiros, O. E., Spinewine, B., Beaubouef, R. T., Sun, T. A. O., Garcia, M. H., and Parker, G.: Bedload transport and bed resistance associated with density and turbidity currents, Sedimentology, 57, 1463–1490, https://doi.org/10.1111/j.1365-3091.2010.01152.x, 2010.
Sømme, T. O., Helland-Hansen, W., Martinsen, O. J., and Thurmond, J. B.: Relationships between morphological and sedimentological parameters in source-to-sink systems: a basis for predicting semi-quantitative characteristics in subsurface systems, Basin Res., 21, 361–387, https://doi.org/10.1111/j.1365-2117.2009.00397.x, 2009.
Soutter, E. L., Bell, D., Cumberpatch, Z. A., Ferguson, R. A., Spychala, Y. T., Kane, I. A., and Eggenhuisen, J. T.: The influence of confining topography orientation on experimental turbidity currents and geological implications, Front. Earth Sci., 8, 1–25, https://doi.org/10.3389/feart.2020.540633, 2021a.
Soutter, E. L., Kane, I. A., Hodgson, D. M., and Flint, S.: The concavity of submarine canyon longitudinal profiles, J. Geophys. Res.-Earth, 126, e2021JF006185, https://doi.org/10.1029/2021JF006185, 2021b.
Spinewine, B., Sequeiros, O. E., Garcia, M. H., Beaubouef, R. T., Sun, T., Savoye, B., and Parker, G.: Experiments on wedge-shaped deep sea sedimentary deposits in minibasins and/or on channel levees emplaced by turbidity currents. Part II. Morphodynamic evolution of the wedge and of the associated bedforms, J. Sediment. Res., 79, 608–628, https://doi.org/10.2110/jsr.2009.065, 2009.
Strak, V., Dominguez, S., Petit, C., Meyer, B., and Loget, N.: Interaction between normal fault slip and erosion on relief evolution: Insights from experimental modelling, Tectonophysics, 513, 1–19, https://doi.org/10.1016/j.tecto.2011.10.005, 2011.
Talling, P. J., Stewart, M. D., Stark, C. P., Gupta, S., and Vincent, S. J.: Regular spacing of drainage outlets from linear fault blocks, Basin Res., 9, 275–302, https://doi.org/10.1046/j.1365-2117.1997.00048.x, 1997.
Vörösmarty, C., Fekete, B. M., Meybeck, M., and Lammers, R. B.: Geomorphometric attributes of the global system of rivers at 30-minute spatial resolution, J. Hydrol., 237, 17–39, https://doi.org/10.1016/S0022-1694(00)00282-1, 2000.
Wan, L., Bianchi, V., Hurter, S., Salles, T., Zhang, Z., and Yuan, X.: Morphological controls on delta-canyon-fan systems: Insights from stratigraphic forward models, Sedimentology, 69, 864–890, https://doi.org/10.1111/sed.12930, 2022a.
Wan, L., Hurter, S., Bianchi, V., Salles, T., Zhang, Z., and Yuan, X.: Combining stratigraphic forward modeling and susceptibility mapping to investigate the origin and evolution of submarine canyons, Geomorphology, 398, 108047, https://doi.org/10.1016/j.geomorph.2021.108047, 2022b.
Weill, P., Lajeunesse, E., Devauchelle, O., Metiver, F., Limare, A., Chauveau, B., and Mouaze, D.: Experimental investigation on self-channelized erosive gravity currents, J. Sediment. Res., 84, 487–498, https://doi.org/10.2110/jsr.2014.41, 2014.